Essay/Term paper: Organic molecules challenge
Essay, term paper, research paper: Information Technology
Free essays available online are good but they will not follow the guidelines of your particular writing assignment. If you need a custom term paper on Information Technology: Organic Molecules Challenge, you can hire a professional writer here to write you a high quality authentic essay. While free essays can be traced by Turnitin (plagiarism detection program), our custom written essays will pass any plagiarism test. Our writing service will save you time and grade.
Organic Molecules Challenge
Silicon's Reign as King of Semiconductors
There is a revolution fomenting in the semiconductor industry. It may take 30
years or more to reach perfection, but when it does the advance may be so great
that today's computers will be little more than calculators compared to what
will come after. The revolution is called molecular electronics, and its goal is
to depose silicon as king of the computer chip and put carbon in its place.
The perpetrators are a few clever chemists trying to use pigment, proteins,
polymers, and other organic molecules to carry out the same task that
microscopic patterns of silicon and metal do now. For years these researchers
worked in secret, mainly at their blackboards, plotting and planning. Now they
are beginning to conduct small forays in the laboratory, and their few successes
to date lead them to believe they were on the right track.
"We have a long way to go before carbon-based electronics replace silicon-based
electronics, but we can see now that we hope to revolutionize computer design
and performance," said Robert R. Birge, a professor of chemistry, Carnegie-
Mellon University, Pittsburgh. "Now it's only a matter of time, hard work, and
some luck before molecular electronics start having a noticeable impact."
Molecular electronics is so named because it uses molecules to act as the
"wires" and "switches" of computer chips. Wires, may someday be replaced by
polymers that conduct electricity, such as polyacetylene and
polyphenylenesulfide. Another candidate might be organometallic compounds such
as porphyrins and phthalocyanines which also conduct electricity. When
crystallized, these flat molecules stack like pancakes, and metal ions in their
centers line up with one another to form a one-dimensional wire.
Many organic molecules can exist in two distinct stable states that differ in
some measurable property and are interconvertable. These could be switches of
molecular electronics. For example, bacteriorhodpsin, a bacterial pigment,
exists in two optical states: one state absorbs green light, the other orange.
Shinning green light on the green-absorbing state converts it into the orange
state and vice versa. Birge and his coworkers have developed high density memory
drives using bacteriorhodopsin.
Although the idea of using organic molecules may seem far-fetched, it happens
every day throughout nature. "Electron transport in photosynthesis one of the
most important energy generating systems in nature, is a real-world example of
what we're trying to do," said Phil Seiden, manager of molecular science, IBM,
Yorkstown Heights, N.Y.
Birge, who heads the Center for Molecular Electronics at Carnegie-Mellon, said
two factors are driving this developing revolution, more speed and less space.
"Semiconductor chip designers are always trying to cram more electronic
components into a smaller space, mostly to make computers faster," he said. "And
they've been quite good at it so far, but they are going to run into trouble
quite soon."
A few years ago, for example, engineers at IBM made history last year when they
built a memory chip with enough transistors to store a million bytes if
information, the megabyte. It came as no big surprise. Nor did it when they came
out with a 16-megabyte chip. Chip designers have been cramming more transistors
into less space since Jack Kilby at Texas Instruments and Robert Noyce at
Fairchild Semiconductor first showed how to put multitudes on electronic
components on a slab of silicon.
But 16 megabytes may be near the end of the road. As bits get smaller and loser
together, "crosstalk" between them tends to degrade their performance. If the
components were pushed any closer they would short circuit. Physical limits have
triumphed over engineering.
That is when chemistry will have its day. Carbon, the element common to all
forms of life, will become the element of computers too. "That is when we see
electronics based on inorganic semiconductors, namely silicon and gallium
arsenide, giving way to electronics based on organic compounds," said Scott E.
Rickert, associate professor of macromolecular science, Case Western Reserve
University, Cleveland, and head of the school's Polymer Microdevice Laboratory.
"As a result," added Rickert, "we could see memory chips store billions of bytes
of information and computers that are thousands times faster. The science of
molecular electronics could revolutionize computer design."
But even if it does not, the research will surely have a major impact on organic
chemistry. "Molecular electronics presents very challenging intellectual
problems on organic chemistry, and when people work on challenging problems they
often come up with remarkable, interesting solutions," said Jonathan S. Lindsey,
assistant professor of chemistry, Carnegie-Mellon University. "Even if the whole
field falls through, we'll still have learned a remarkable amount more about
organic compounds and their physical interactions than we know now. That's why I
don't have any qualms about pursuing this research."
Moreover, many believe that industries will benefit regardless of whether an
organic-based computer chip is ever built. For example, Lindsey is developing an
automated system, as well as the chemistry to go along with it, for synthesizing
complex organic compounds analogous to the systems now available for peptide and
nucleotide synthesis. And Rickert is using technology he developed foe molecular
electronic applications to make gas sensors that are both a thousand times
faster and more sensitive than conventional sensors.
For now, the molecular electronics revolution is in the formative stage, and
most of the investigations are still basic more than applied. One problem with
which researchers are beginning to come to grips, though, is determining the
kinds if molecules needed to make the transistors and other electronic
components that will go into the molecular electronic devices, Some of the
molecules are like bacteriorhodopsin in that their two states flip back and
forth when exposed to wavelengths of light. These molecules would be the
equivalent of an optical switch on which on state is on and the other state is
off. Optical switches have been difficult to make from standard semiconductors.
bacteriorhodopsin is the light-harvested pigment of purple bacteria living in
salt marshes outside San Francisco. The compound consists of a pigment core
surrounded by a protein that stabilizes the pigment. Birge has capitalized on
the clear cut distinction between the two states of bacteriorhodopsin to make
readable-write able optical memory devices. Laser disks, are read-only optical
memory devices, once encoded the data cannot be changed.
Birge has been able to form a thin film of bacteriorhodopsin on quartz plates
that can then be used as optical memory disks. The film consists of a thousand
one-molecule thick layers deposited one layer at a time using the Langmuir-
Blodgett technique. A quartz plate is dipped into water whose surface is covered
with bacteriorhodopsin. When the plate is withdrawn at a certain speed, a
monolayer of rhodopsin adheres to the plate with all the molecules oriented in
the same direction. Repeating this process deposits a second layer, then a third,
and so on.
Information is stored by assigning 0 to the green state and 1 to the orange
state. Miniature lasers of the type use din fiber optic communications devices
are used to switch between the two states.
Irradiating the disk with a green laser converts the green state to the orange
state, storing a 1. resetting the bit is accomplished by irradiating the same
small area of the dusk with a red laser. Data stored on the disk are read by
using both lasers. The disk would be scanned with the red laser and any bit with
a value 1 would be reset using the green laser.
This is analogous to the way in which both magnetic and electrical memories are
read today, but with one important difference: "Because the two states take only
five picoseconds (five trillionths of a second) to flip back and forth,
information storage and retrieval are much faster than anything you could ever
do magnetically or electrically," explained Birge.
In theory, each pigment molecule could store one bit of information. In practice,
however approximately 100,000 molecules are sued. The laser beam as a diameter
if approximately 10 molecules and penetrates through the 1,000 molecule think
layer. Although this reduces the amount of information that can be stored on
each disk, it does provide fidelity though redundancy.
"We can have half the molecules or more in a disk fall apart and there would
still be enough excited by the laser at each spot to provide accurate data
storage," said Birge. And even using 100,000 molecules per data bit, an old 5.25
inch floppy disk could store well over 500 megabytes of data.
One drawback to this system is that the bacteriorhodopsin's two states are only
stable at liquid nitrogen temperatures, -192¡C. But Birge does not see this as
anything more than a short term problem. "We're now using genetic engineering to
modify the protein part of the molecule so that it will stabilize the two states
at room temperature," he said. "Based in outstanding work, we don't think this
will be a problem."
Faster, higher-density disk storage is a laudable goal, but the big stakes are
in improving on semiconductor components. Birge, for example, is developing a
random access chip using the bacteriorhodopsin system. Instead of having
millions of transistors wired together on a slab of silicon, there would be
millions of tiny lasers pointed at a film of bacteriorhodopsin. "These RAM chips
would actually be a little bigger than what we have," he said, "but they would
still be 1,000 times faster because the molecular components work so much faster
than ones made of semiconductor materials."
Recently, Theodre O. Poehler, director of research, John Hopkin's Applied
Physics Laboratory, Laurel, Md., and Richard S. Potember, a senior chemist there,
built a working four-byte RAM chip using molecular charge-transfer system. Four
bytes may seem crude compared to the million-byte chip built by IBM, but the
first semiconductor chip, built by Texas Instruments' Kilby in 1959, was also
crude compared to today's chips.
Poehler and Potember's system also uses laser light to activate the molecular
switches, but the chemistry is much different than Birge's. In the Carnegie-
Mellon system, light causes an electron on the bacteriorhodopsin to move into a
higher energy level within the same molecule. This changes its absorption
spectrum. In the Hopkin's system, light causes an electron to transfer between
two different molecules, one called an electron donor, the other an electron
acceptor. This is known as a charge-transfer reaction, and the researchers in
several laboratories are designing devices using this type of molecular switch.
In their system, Poehler and Potember use compounds formed form either copper or
silver- the electron donor-and the tetracyaboquinodimethane (TCNQ) or various
derivatives-the electron acceptor. The researchers first deposit the metal onto
some substrate-it could be either a silicon or plastic slab. Next, they deposit
a solution of the organic electron acceptor onto the metal and heat it gently,
causing a reaction to occur and evaporating the solvent.
In the equilibrium state between these two molecular components, an electron is
transferred from copper to TCNQ, forming a positive metal ion and a negative
TCNQ ion. Irradiating this complex with light from an argon laser causes the
reverse reaction to occur, forming neutral metal and neutral TCNQ.
Two measurable changed accompany this reaction. One is that the laser-lit area
changes color from blue to a pale yellow if the metal is copper or from violet
if it is silver. This change is easily detected using the same or another laser.
Thus, metal TCNQ films, like those made from bacteriorhodopsin, could serve as
optical memory storage devices. Poehler said that they have already built
several such devices and are now testing their performance. They work at room
temperature.
The other change that occurs, however, is more like those that take place on
standard microelectronics switches. When an electric field id applied to the
organometallic film, it becomes conducting in the irradiated area, just as a
semiconductor does when an electric field is applied to it.
Erasing a data or closing the switch is accomplished using any low-intensity
laser, including carbon dioxide, neodymium yttrium aluminum garnet, or gallium
arsenide devices. The tiny amount of heat generated by the laser beam causes the
metal and TCNQ to return to their equilibrium, non-conducting state. Turning off
the applied voltage also returns the system to its non-conducting state.
The Hoptkins researchers found they could tailor the on/off behavior of this
system by changing the electron acceptor. Using relative weak electron acceptors,
such as dimethoxy-TCNQ, produced organometallic films with a very sharp on/off
behavior. But of a strong electron acceptor such as tetrafluoro-TCNQ is used,
the film remains conductive even when the applied field is removed. This effect
can last from several minutes to several days; the stronger the electron
acceptor, the longer the memory effect.
Poehler and his colleagues are now working to optimize the electrical and
optical behavior of these materials. They have found, for example, that films
made with copper last longer than those made of silver. In addition, they are
testing various substrates and coatings to further stabilize these systems. "We
know the system works," Poehler said. "Now we're trying to develop it into a
system that will work in microelectronics applications."
At Case Wester Rickert is also trying to make good organic chemistry and turn it
into something workable in microelectronics. He and his coworkers have found
that using Langmuir-Blodgett techniques they can make polymer films actually
look like and behave like metal foils. "The polymer molecules are arranged in a
very regular, ordered array, as if they were crystalline," said Rickert.
These foils, made from polymers such as polyvinylstearate, behave much as metal
oxide films do in standard semiconductor devices. but transistors made with the
organic foils are 20 percent faster than their inorganic counterparts, and
require much less energy to make and process. Early in 1986, Rickert made a
discovery about these films that could have a major impact on the chemical
industry long before any aspect of molecular electronics. "the electrical
behavior of these foils is very sensitive to environmental changes such as
temperature, pressure, humidity and chemical composition," he said. "As a result,
they make very good chemical sensors, better than any sensor yet developed."
He has been able to develop an integrated sensor that to date can measure parts
per billion concentrations of nitrogen oxides, carbon dioxide, oxygen, and
ammonia. Moreover, it can measure all four simultaneously.
Response times for the new "supersniffer," as Rickert calls the sensor, are in
the millisecond range, compared to tens of seconds for standard gas sensors,
Recovery times are faster too; under five seconds compared to minutes or hours.
The Case Western team is now using polymer foils as electrochemical and
biochemical detectors.
In spite of such successes, molecular electronics researchers point out that
MEDs will never replace totally those made of silicon and other inorganic
semiconductors. "Molecular electronics will never make silicon technology
obsolete," said Carnegie-Mellon's Birge. "The lasers we will need, for example,
will probably be built from gallium arsenide crystals on silicon wafers.
"But molecular electronic devices will replace many of those now made with
silicon and the combination of the two technologies should revolutionize
computer design and function."
Other sample model essays:
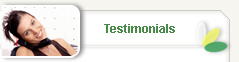

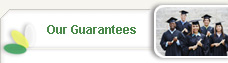

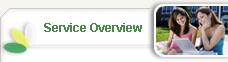

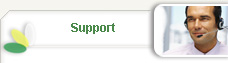

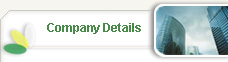
